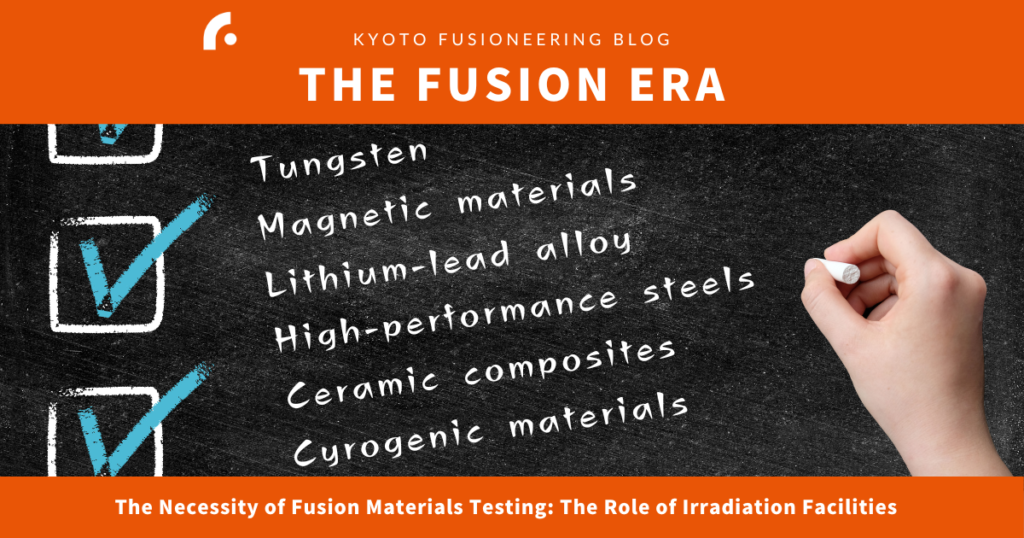
Dear Fusion Enthusiasts,
In this blog post, let’s take a journey into the heart of fusion Research and Development (R&D): materials for fusion. The road to commercially practical fusion energy comes with challenges, and a key hurdle is developing materials that can endure the extreme conditions inside a fusion plant. We’ll explore the challenges posed by these materials, shed light on the crucial role of irradiation testing, and keep you in the loop with the latest in fusion materials research. Together, let’s uncover the potential of fusion energy!
Challenges of Fusion Materials
Most of fusion environments will include high thermal loads, high-energy neutron bombardment, plasma-material interactions, substantial presence of tritium, and corrosion. To develop materials for such severe operational conditions, researchers must first find the desired properties, such as radiation resistance, high melting point, low sputtering yield, low activation, and cost effectiveness. Materials for plasma-facing components have the most stringent requirements.
Once the desired properties have been found and quantified, candidate materials must be tested to decide if they meet the required criteria. Key tests include irradiation testing and activation measurement, hot temperature and heat flux testing, plasma exposure, and mechanical, corrosion testing, and any combination of these effects. Irradiation testing is an essential aspect and the bottleneck of materials development for fusion energy applications. It requires exposing samples to high-energy particles, most importantly neutrons, at enough fluence to evaluate their degradation under the extreme conditions expected in a fusion plant. The degradation of the material is quantified in terms of “displacements per atom” (dpa) and is due to collision cascades within the material microstructure. While ITER (International Thermonuclear Experimental Reactor) will have less than 3 dpa over its lifetime, in a fusion power plant the damage is expected to have 15 dpa per year of operation and we expect to run a few years before we need to replace the materials.
Objectives of Irradiation Testing
The primary aims of irradiation testing campaigns are to create a database to benchmark the radiation responses of materials in collaboration with computational material science and to generate irradiation data to support the design, licensing, construction, and safe operation of a fusion power plant. From a safety point of view, regulators will request the qualification of these materials.
At present, only limited neutron irradiation testing can currently be achieved with research fission reactors and spallation neutron sources. The former can produce neutrons with an average energy around 1-2 MeV, but the generation of α-particles (helium production, measured in units of appm/dpa) is insufficient to replicate a fusion environment. Existing spallation sources (with Hg, Pb and U as proton targets) can supply a wide energy spectrum but tend to generate light transmuted nuclei due to the hundreds MeV tails, affecting the target’s mechanical properties. The alternative is to use ion irradiation to simulate the effects of damage and transmutation products, but the correlation is not exact. Only micron-scale regions of samples can be irradiated, so macro-scale (bulk) properties cannot be examined. One example of an ion irradiation facility is the DUET facility at Kyoto University. Kyoto Fusioneering has also irradiated material samples at the Surrey Ion Beam Centre in collaboration with the Material Research Facility at UKAEA (United Kingdom Atomic Energy Authority).
International Research Organisations and Laboratories
Several international research organizations and laboratories are actively engaged in advancing fusion technology through materials testing and its irradiation facilities. These organizations include Oak Ridge National Laboratory (ORNL) and its High Flux Isotope Reactor (HFIR) and the Spallation Neutron Source (SNS); Japan Atomic Energy Agency (JAEA) and its Japan Materials Testing Reactor (JMTR) and the Experimental Fast Reactor Jōyō; Culham Centre for Fusion Energy (CCFE) and the Materials Research Facility (MRF); Idaho National Laboratory (INL) and its Tritium Plasma Experiment (TPE); Naka Fusion Institute (NFI) and its Large Helical Device (LHD); Centre for Energy, Environmental and Technological Research (CIEMAT); Jülich Centre for Neutron Science (JCNS); Belgian Nuclear Research Centre (SCK CEN) and its BR2 Reactor; China Institute of Atomic Energy (CIAE) and its China Advanced Research Reactor (CARR). Additionally, there exist other facilities and research centres not detailed here. Following irradiation, various methods exist to see microstructural damage, including Scanning Electron Microscopy (SEM), Transmission Electron Microscopy (TEM), X-ray Diffraction (XRD), and Positron Annihilation Spectroscopy (PAS).
New Endeavours toward advancing Irradiation Testing
The most promising fusion reaction is deuterium-tritium (DT) which generates 14 MeV neutrons. The number of neutrons produced in a fusion power plant is measured in terms of flux and will be in the order of 1018 m-2s-1. Despite the enormous effort made by the experiments above, the damage mechanisms in materials exposed to fusion neutrons differ significantly and experience has led us to an important conclusion: only fusion-like neutrons can be used to accurately decide materials performance in a fusion device. This is the motivation for projects such as the Fusion Prototypic Neutron Source (FPNS) in the U.S. and IFMIF-DONES (International Fusion Materials Irradiation Facility-DEMO Oriented Neutron Source) in Spain and others similar projects.
In the deuteron-lithium stripping reaction Li(d, n) exploited for IFMIF-DONES, protons can be “stripped” off leaving the neutrons to proceed in the forward direction with the velocity of the incident deuteron. This reaction will be able to supply an adequate fusion neutron spectrum capable of producing 20-50 dpa per year in the high flux region at different controlled temperatures up to 1000oC. Its construction has already started in Escúzar (Granada, Spain) and is expected to be operational in 10 years. It is expected to supply correct material characterization data needed for the safe design, construction, and regulatory licensing of a fusion power facility.
Finally, the need for a fusion-like neutron source requires parallel efforts to develop remote-handling technologies and to build efficient hot cells. These parallel efforts will be essential to enable correct and safe examination and analysis of the irradiated materials.
Kyoto Fusioneering’s latest approach
Kyoto Fusioneering (KF) has signed a Memorandum of Understanding with IFMIF-DONES to accelerate its development and to contribute with KF’s materials knowledge and unique network. Also, KF is developing a fusion-grade interface-less SiCf/SiC material (silicon carbide reinforced by silicon carbide fibres) in collaboration with Kyoto University. Samples fabricated by KF and irradiated in the Surrey Ion Beam Centre in collaboration with UKAEA have shown stability against low dpa irradiation under conditions simulating Si-burnup. Earlier this year, a collaboration agreement was signed between UKAEA and Kyoto Fusioneering, to advance materials development for commercial fusion energy.
The development of materials that can withstand the extreme conditions inside a fusion plant is essential for the commercialization of fusion energy. By understanding how materials respond to radiation and developing materials with the required properties, scientists can pave the way for a safe, reliable, and environmentally friendly fusion energy source.
“In spite of the considerable efforts that have been made in modelling irradiation damage in fusion materials, I believe ‘theory’ alone cannot further down select the best material choice. We need fusion-like irradiation conditions to address the material’s challenges that are still unsolved.”
Mario Oliver, Fusion Engineer, Materials Team, Kyoto Fusioneering